The 2015 publication of ANSI/ASHRAE 188-2015, Legionellosis: Risk Management for Building Water Systems, fundamentally changed how Legionnaires’ disease is handled in the United States. In 2017 the Centers for Medicare and Medicaid Services mandated that all hospitals and long-term care facilities implement a water management plan to reduce the risk of Legionnaires’ disease, referencing ANSI/ASHRAE 188 as the basis for such plans. This fundamentally altered the standard of care for preventing diseases caused by Legionella and other waterborne pathogens in healthcare settings. The amount of data these water management programs generate quickly becomes overwhelming. The approaches often used to manage this mountain of data are to collect less data or to only evaluate data points in isolation, both of which ultimately hinder our ability to understand the condition of water systems.
ANSI/ASHRAE 188 provides little guidance on sampling and interpreting measurement results, and provides no guidance at all on how to plan and carry out a Legionella risk assessment. While guidance exists on interpreting some of the parameters used to control Legionella growth, a practical tool to assess the combined efficacy of multiple parameters has not previously been available.
To address this challenge, I developed a multivariate model to evaluate the combined effectiveness of control measures against Legionella and other waterborne pathogens in building potable water systems. The model allows users to evaluate a water system’s susceptibility to Legionella amplification and to prioritize corrective actions or improvements for systems found to be at high risk.
Interpretation of water sampling results for Legionella is beyond the scope of the model but can be used to validate the effectiveness of control measures. Table 3.1 of the AIHA guideline “Recognition, Evaluation, and Control of Legionella in Building Water Systems” offers criteria for interpreting the results of Legionella water sampling to determine whether amplification is occurring.
RESOURCES
AIHA: Recognition, Evaluation, and Control of Legionella in Building Water Systems (2015).
American Society of Heating, Refrigerating and Air-Conditioning Engineers: ANSI/ASHRAE Standard 188-2018, Legionellosis: Risk Management for Building Water Systems (August 2018).
Annals of Clinical and Laboratory Science: “The Effect of Drying, Heat, and pH on the Survival of Legionella pneumophila” (May 1987).
Applied and Environmental Microbiology: “Factors Influencing Survival of Legionella pneumophila Serotype 1 in Hot Spring Water and Tap Water” (May 2003).
ASHRAE: Standard Guideline 12-2000, Minimizing the Risk of Legionellosis Associated with Building Water Systems (February 2000).
ASTM International: D5952 – 08, Standard Guide for the Inspection of Water Systems for Legionella and the Investigation of Possible Outbreaks of Legionellosis (Legionnaires’ Disease or Pontiac Fever) (July 2008).
BuildingGreen.com: “The Surprising Connection Between Water Conservation and Deadly Infections” (December 2014).
CDC: “Sampling Procedure and Potential Sampling Sites: Protocol for Collecting Environmental Samples for Legionella Culture During a Cluster or Outbreak Investigation or When Cases of Disease May Be Associated with a Facility” (June 2015).
CDC: “Toolkit for Developing a Water Management Program to Reduce Legionella Growth & Spread in Buildings: A Practical Guide to Implementing Industry Standards” (PDF, June 2017).
CDC: “Vital Signs: Deficiencies in Environmental Control Identified in Outbreaks of Legionnaires’ Disease—North America, 2000–2014” (June 2016).
Centers for Medicare & Medicaid Services: “Requirement to Reduce Legionella Risk in Healthcare Facility Water Systems to Prevent Cases and Outbreaks of Legionnaires’ Disease (LD)” (July 2018).
Clinical Microbiology Reviews: “Legionella and Legionnaires' Disease: 25 Years of Investigation” (July 2002).
Current Microbiology: “Measure of Legionella pneumophila Activity in Situ” (March 1981).
Environmental Science: Water Research & Technology: “Critical Review of Mathematical Approaches for Quantitative Microbial Risk Assessment (QMRA) of Legionella in Engineered Water Systems: Research Gaps and a New Framework” (March 2016).
EPA: “Effects of Water Age on Distribution System Water Quality” (PDF, August 2002).
EPA: “Health Risks from Microbial Growth and Biofilms in Drinking Water Distribution Systems” (June 2002).
EPA: “National Primary Drinking Water Regulations: Stage 2 Disinfectants and Disinfection Byproducts Rule” (PDF, January 2006).
EPA: “Technologies for Legionella Control in Premise Plumbing Systems: Scientific Literature Review” (September 2016).
EPA: “Water Conservation at EPA.”
Federal Register: “National Primary Drinking Water Regulations: Disinfectants and Disinfection Byproducts” (PDF, December 1998).
Holland Water: “The Efficacy of Various Disinfection Methods against Legionella pneumophila in Water Systems: A Literature Review” (PDF).
International Biodeterioration & Biodegradation: “Environmental Factors Affecting the Colonisation of Cooling Towers by Legionella spp. in South Australia” (1993).
National Research Council: Drinking Water Distribution Systems: Assessing and Reducing Risks (2006).
Water Research: “Impact of Advanced Water Conservation Features and New Copper Pipe on Rapid Chloramine Decay and Microbial Regrowth” (March 2012).
Water Science and Technology: “Legionella and Public Water Supplies” (November 1988).
World Health Organization: “Legionella and the Prevention of Legionellosis” (2007).
BACKGROUND
Regulatory pressures to reduce energy and water consumption, and to limit exposures to potentially carcinogenic disinfectant byproducts, coupled with an aging potable water distribution infrastructure, have preceded record rates of Legionnaires’ disease across the United States (see Figure 1). Between 2015 and 2018, publications from ASHRAE, CDC, AIHA, and the Centers for Medicare and Medicaid Services reversed past recommendations regarding waterborne pathogens and began advocating a proactive approach to prevent Legionella amplification. These changes focused on implementing water management programs in healthcare operations and other “at-risk” facilities, and include recommendations to periodically measure water temperatures, disinfectant or oxidant levels, and other parameters believed to influence the amplification of Legionella in building water systems. Some publications direct that a risk assessment be performed to identify where Legionella and other waterborne pathogens could grow and spread in the facility water system. However, only the AIHA guideline defines the parameters of a Legionella risk assessment and provides a framework for how to plan, conduct, and interpret the findings.
LEGIONELLA SOURCE RISK ASSESSMENT
The primary goal of a Legionella risk assessment is to identify potential amplification sites and transmission sources to minimize risks of exposure. In its “Toolkit for Developing a Water Management Program to Reduce Legionella Growth & Spread in Buildings,” CDC has recognized that for Legionella to pose a health risk, it must first grow or amplify in number. The sites where Legionella amplifies in potable water systems, water heaters, cooling towers, or other constructed water sources pose a risk of exposure that may result in Legionnaires’ disease. Early recognition and detection of Legionella amplification sites can be accomplished through a rigorous source risk assessment, which is described in chapter 3 of the AIHA guideline.
After performing an initial inventory of water sources and systems, the industrial hygienist (or other competent professional, as defined in AIHA’s Legionella guideline) needs to assess the building water systems and characterize their susceptibility to Legionella colonization and amplification. This is often the most challenging phase of the risk assessment because few tools are available to analyze the multiple variables, inputs, and measurement parameters. This subjective approach promotes a reliance on the assessor’s professional judgment, which can result in errors of interpretation.
A Legionella source risk assessment performed as part of a routine or initial risk assessment, where no cases of Legionnaires’ disease have been identified, should assess all potential sources. For most buildings this includes potable, non-potable, and industrial water systems that can generate aerosols. The model presented in this article combines a wide variety of data, observations, and measurements into a numerical score that characterizes the overall effectiveness of control measures (Ceff) to inhibit Legionella amplification. A low Ceff score indicates less effective control and, therefore, conditions more conducive to Legionella amplification. As such, the model enables the assessor to rely upon an objective and reproducible process to assess Legionella risk allowing for more consistent and data-driven recommendations.
DATA COLLECTION AND INTERPRETATION
Guidance on the data to collect as part of a Legionella source risk assessment is highly varied and sometimes vague. The percentage of distal points to sample and how to interpret the results of sampling depends on how the water system is constructed and must be determined by each competent professional. The model allows for synthesis of all available data, measurements, and observations into a composite characterization of the water system.
The model integrates multiple Legionella control measures into a single metric of effectiveness. The model generates a conservative estimate of the “effectiveness of control” score (Ceff score) using as few as two measurements, water temperature (T) and oxidant concentration ([Ox]). The model can also calculate a more robust estimate of Ceff using up to eight parameters. The three components of the model are temperature function (Tf), oxidant concentration function ([Ox]f), and water age function (Wage).
Water temperature can either encourage or inhibit Legionella amplification within a water system. Legionella survives but typically does not amplify at temperatures below 70 F (21 C). Above 130 F (54 C) Legionella begins to die and its amplification is severely inhibited. The ideal temperature range for Legionella amplification has been reported to be 90–108 F (32–42 C) with a mean of 99 F (37 C). For ease of calculation, 100 F (37.8 C) is used as the midpoint estimate of least effective control. The first function of the model is calculated by subtracting the measured temperature from 100 F and dividing the absolute value of the difference by 10:
ADVERTISEMENT
CLOSE
Figure 1. Number of Legionnaires’ Disease Cases Reported Annually
Tap on the figure to open a larger version in your browser.
AIHA Guideline Undergoing Revision
Since the May 2015 publication of AIHA’s guideline “Recognition, Evaluation, and Control of Legionella in Building Water Systems,” several other guidelines, standards, mandates, and regulations have been issued. To ensure the AIHA guideline on Legionella remains relevant, AIHA’s Indoor Environmental Quality Committee has begun the process of updating and revising the guideline. The anticipated completion date is early 2020.
Like water temperature, oxidant concentrations can either encourage or inhibit Legionella amplification. For free chlorine oxidant, the influence of elevated pH must also be considered. The pH function (FpH) is calculated (within the range of pH 6.5 and 8.5), and the resulting effect on oxidant concentration is integrated into the model.
The type of oxidant (free chlorine, chlorine dioxide, or monochloramine) and the effective concentration needed to inhibit Legionella amplification are accounted for in the model’s oxidant type function (OTF). Maintaining free chlorine concentrations above 2.0 mg/L (ppm), at a pH of 7.2 to 7.8, has been reported to continuously control Legionella growth or prevent its amplification. Oxidant type function values (OTF) for free chlorine (1.0), chlorine dioxide (2.5), and monochloramine (0.5) are applied to the calculation:
The final factor used in the model to assess the water system’s resistance to Legionella amplification is water age (Wage). Infrequently used or stagnant water has been associated with increased risk of biofilm development and Legionella amplification. Frequent use, maintenance of inhibitory temperature and oxidant levels at distal fixtures, and short branch runs between circulating water and points of use are factors that help to reduce water age.
The following measurements and observations are used to assess water age:
- T1: water temperature (°F), first draw
- T2: water temperature (°F), flushed until temperature stabilizes
- [Ox]1: water oxidant concentration (ppm), first draw
- [Ox]2: water oxidant concentration (ppm), flushed until temperature stabilizes
- ΔTime: time function (minutes), the amount of time it takes to flush water until the temperature stabilizes
- F: frequency of use (scale of 1 to 5), determined through interview or observation, not parameter measurement
The scale for F is:
- 5 = Several times every day
- 4 = Five to seven times per week or once per day
- 3 = Three times per week
- 2 = Twice per week
- 1 = Once per week or less frequently
- 0 = Unknown
When data or observations are available to inform the assessment of these factors, the model can be refined to indicate an increased Ceff score. Without data to calculate the water age function, the base model assumes that water age is unfavorable or ineffective at inhibiting Legionella amplification:
The complete model to calculate the Ceff is:
Scores calculated by the model can range from zero to six. The score is a calculation of a potable water system’s resistance to Legionella amplification. With as little as two measurements (temperature and oxidant concentration) and one observation (oxidant type), a conservative score can be calculated. A greater number of distal points included in the risk assessment along with additional measurements and observations collected at each point generates a more robust score that is more reliable and repeatable. Explanations of Ceff scores appear in Table 1.
Table 1. Interpreting Ceff Scores
PRIORITIZING ACTIONS
Aggregating Ceff scores to assess a whole system, such as the hot water system, can provide a more useful and relevant characterization. It is important not to combine scores of independent systems: the resulting aggregate score would not reflect either system and could obscure a poorly controlled system due to bias introduced from a well-controlled system.
Aggregated Ceff scores can be used to initially evaluate various water systems as part of a Legionella source risk assessment and be tracked over time. Water systems and sources with low Ceff scores may be prioritized for further assessment, corrective measures, Legionella-specific sampling, or all of the above. The variability of Ceff scores throughout a system can indicate areas of deficiency or a system that is inherently at greater risk. Data outliers from the mass of data should prompt investigation of the underlying reason.
Examples of corrective actions may include increasing hot water storage and circulating temperatures, removing dead legs, or increasing the flushing frequency of effective dead legs. Other changes to water systems to reduce the risk of Legionella growth can include re-routing cold-water lines to prevent warming; increasing oxidant concentrations through increased water usage or adding supplemental disinfectants; or implementing preventative maintenance of key system components.
A DEFENSE AGAINST LEGIONELLA
Each control factor is essentially a “brick” in the defensive “wall” against Legionella growth in a potable water system. The size and strength of each brick adds to the overall effectiveness of the wall to prevent Legionella amplification. An effective wall can be built with a single brick if it is large enough, but it may not be as resilient as a wall built with multiple defensive components.
This analogy forms the basis of the scoring system developed to assess the risk of Legionella amplification. While a low Ceff score does not definitively predict Legionella amplification, it does mean that the water source is more susceptible to amplification. A high Ceff score, especially one that trends over time, is a strong indicator that control measures, if maintained, are effective at preventing Legionella growth. Little published guidance is available regarding prioritization of control measures for preventing Legionella amplification. This model provides a data-driven method to assist competent professionals and water management teams with decisions about allocating time, personnel, and resources to manage water systems for Legionella.
J. DAVID KRAUSE, PhD, MSPH, CIH, is the national practice leader in Toxicology and Risk Assessment for Forensic Analytical Consulting Services (FACS) in Tallahassee, Fla. From 2008 to 2011, he served as the state toxicologist for the Florida Department of Health. He co-authored the 2009 “Guidelines for the Surveillance, Investigation, and Control of Legionnaires’ Disease in Florida” and the 2015 AIHA “Guideline for the Recognition, Evaluation, and Control of Legionella in Building Water Systems.”
Send feedback to The Synergist.
Legionella pneumophila artwork.
royaltystockphoto/Getty Images
Legionnaires’ Disease Cases Continue to Rise
Despite the publication of numerous standards, mandates, and regulations in response to increasing rates of Legionnaires’ disease across the U.S., rates of disease continue to rise. Since 2002, the number of Legionnaires’ disease cases has increased five-fold. The total number of cases reported to the CDC in 2018 (8,356) exceeded the number reported in 2017 (7,458), an increase of 12 percent.
Most healthcare facilities were mandated to perform risk assessments for Legionella sources and implement water management plans to prevent disease caused by Legionella and other waterborne pathogens in June 2017. However, these efforts have not yet resulted in abatement of the upward trend in disease. Regulations requiring cooling tower operators in New York City and New Orleans to register, maintain, clean, test, and report Legionella contamination have also been enacted. In light of new requirements and an emerging standard of care for operators of buildings and cooling towers to prevent conditions that lead to outbreaks of Legionnaires’ disease, the ongoing increase in cases should prompt all stakeholders to reevaluate their programs and operations. The influence of external factors such as climate change, rainfall amounts, disinfectant levels in supply water, and degrading equipment and infrastructure has not yet been fully assessed. Industrial hygienists should be prepared to assist communities in addressing this growing and complex issue.
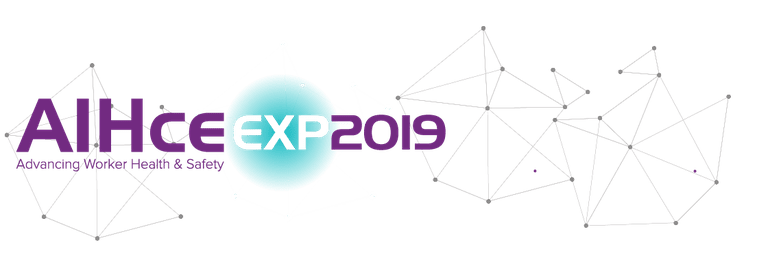
Tap on the equation to open a larger version in your browser.
A Hazard Analysis Tool for Data Interpretation
BY J. DAVID KRAUSE
PERFORMING Legionella SOURCE RISK ASSESSMENTS
Although the print version of The Synergist indicated The IAQ Investigator's Guide, 3rd edition, was already published, it isn't quite ready yet. We will be sure to let readers know when the Guide is available for purchase in the AIHA Marketplace.
My apologies for the error.
- Ed Rutkowski, Synergist editor
Disadvantages of being unacclimatized:
- Readily show signs of heat stress when exposed to hot environments.
- Difficulty replacing all of the water lost in sweat.
- Failure to replace the water lost will slow or prevent acclimatization.
- Increased sweating efficiency (earlier onset of sweating, greater sweat production, and reduced electrolyte loss in sweat).
- Stabilization of the circulation.
- Work is performed with lower core temperature and heart rate.
- Increased skin blood flow at a given core temperature.
- Gradually increase exposure time in hot environmental conditions over a period of 7 to 14 days.
- For new workers, the schedule should be no more than 20% of the usual duration of work in the hot environment on day 1 and a no more than 20% increase on each additional day.
- For workers who have had previous experience with the job, the acclimatization regimen should be no more than 50% of the usual duration of work in the hot environment on day 1, 60% on day 2, 80% on day 3, and 100% on day 4.
- The time required for non–physically fit individuals to develop acclimatization is about 50% greater than for the physically fit.
- Relative to the initial level of physical fitness and the total heat stress experienced by the individual.
- Can be maintained for a few days of non-heat exposure.
- Absence from work in the heat for a week or more results in a significant loss in the beneficial adaptations leading to an increase likelihood of acute dehydration, illness, or fatigue.
- Can be regained in 2 to 3 days upon return to a hot job.
- Appears to be better maintained by those who are physically fit.
- Seasonal shifts in temperatures may result in difficulties.
- Working in hot, humid environments provides adaptive benefits that also apply in hot, desert environments, and vice versa.
- Air conditioning will not affect acclimatization.
Acclimatization in Workers