DEPARTMENTS
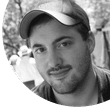
INSTRUMENTATION
SPENCER PIZZANI, CIH, is the occupational health manager for PepsiCo Global EHS.
Previous installments in this series discuss carbon monoxide exposures to foundry crane operators and the use of four-gas personnel monitors in surface mine blasting.
Send feedback to The Synergist.
Practical Examples of Alarm-Setting Guidance, Part 3
BY SPENCER PIZZANI
Setting alarms for direct-reading instruments (DRI) can be the most complex part of implementing a real-time detection system (RTDS). A new document available from AIHA (PDF) provides practical guidance for setting alarms. This process includes five key steps to determine the appropriate alarm settings based on a systematic analysis of signaling context (see Table 1).
The AIHA RTDS Committee is pleased to partner with The Synergist to provide this third practical example of alarm-setting guidance in action to reinforce practical application. The following scenario concerns the use of a fixed carbon dioxide measurement system in a brewery.
STEP 1: ESTABLISH STATEMENT OF PURPOSE
A regional brewery with four production lines produced brewed beverages to fill aluminum cans. The brewing process releases carbon dioxide (CO2) into the indoor atmosphere. The statement of purpose for the CO2 measurement system was to alert workers when conditions within the working area exceed exposure limits.
STEP 2: SELECT OBJECTIVES
CO2 is a colorless, odorless gas with a variety of health and safety concerns. The ACGIH threshold limit value (TLV) is based on asphyxia, but CO2 exposure may also be associated with various neurological health effects and may contribute to safety incidents as a secondary effect. According to the AIHA alarm-setting guidance, these exposure risks and the stated purpose of detection classify CO2 as a Designation C agent, which indicates that it requires combined safety and health monitoring. The objective of monitoring was to prompt workers to relocate outside of the CO2-enriched atmosphere to mitigate exposed dose.
STEP 3: SELECT DEVICES AND DETERMINE FITNESS FOR PURPOSE
A review of CO2 detection options determined that a permanently installed (that is, fixed) gas detection system that could interface with facility ventilation was desirable and available from several manufacturers. However, capabilities differed between designs, and most manufacturers offered several models. The evaluation for fitness considered the complete system, not just the sensors. The outputs and relays needed to be capable of controlling peripheral equipment such as fans and alarms. The routine use of personal monitors could not provide this capability, so a stationary system was necessary.
Importantly, the evaluation considered whether the measurement system alarms were limited to real-time (that is, peak) readings or included STEL (short-term exposure limit) and TWA (time-weighted average) alarm calculations. The control panel was determined to be capable of both instantaneous alarms and time-delayed alarms based on a programmed period of up to two hours. The proposed system architecture allowed for interface with a cloud database that would receive and retain data logs for future evaluation.
Other critical factors considered were the effects of ambient conditions such as temperature and humidity, potentially low oxygen levels, and the presence of potential interferants such as ethanol. The AIHA Standardized Equipment Specification Sheet (PDF) was used to help clarify sensor performance capabilities.
Because of their substance-specificity, stability, operability over a wide range of temperature and humidity conditions, durability, and cost, nondispersive infrared (NDIR) sensors are almost universally preferred for CO2 detection. CO2 sensors are designed for use in specific ranges and resolutions and are capable of measurement in either ppm or percent volume increments.
For reference, the TLV-TWA is 5,000 ppm for CO2, with a TLV-STEL of 30,000 ppm. However, the brewery was in British Columbia, Canada, which has a provincial regulatory STEL of 15,000 ppm as promulgated by WorkSafeBC (WSBC). The brewery did not anticipate extended work shifts.
The brewery considered two instruments with the range and resolution of the CO2 sensors. The first instrument expressed readings in 1.0 percent volume (10,000 ppm) increments over a full measurement range of 0–7.5 percent volume (75,000 ppm). The second instrument expressed readings in 500 ppm increments over a full range of 0–50,000 ppm. The brewery selected the second instrument because the limit of quantitation (LOQ) for the first option (1.0 percent volume or 10,000 ppm intervals) was too high for the health objectives identified for Designation C detection.
A final consideration was the placement of the sensors and transmitters for the system. The sensors needed to be close enough to workers’ positions to reasonably represent breathing zone concentrations. The transmitters were placed near workstations but away from any direct gas streams that might impact the validity of measurements.
STEP 4: ESTABLISH ALARM SETPOINT AND RESPONSE PROCESS
The brewery determined that the primary requirement was to comply with the WSBC STEL of 15,000 ppm. However, management recognized that the concentration of CO2 in the production area was highly variable and wanted to prompt both mechanical and employee action before the STEL was exceeded.
Therefore, the ventilation fan was set to increase to maximum airflow when the room reached an instantaneous concentration of 12,000 ppm. Historical ambient monitoring showed that increases in room concentrations above this number often led to potential exposures over the WSBC STEL. At 12,000 ppm, the system activates an amber-colored warning strobe but not the alarm horn signaling evacuation. The fan continues to run at maximum airflow for 15 minutes after the initial alarm level clears.
The system is programmed to flash red warning lights and sound high-intensity horns (at a carefully determined 3:1 signal-to-noise ratio above background noise) when the average concentration reaches 15,000 ppm over the most recent 10-minute interval (a shorter duration than the WSBC STEL). This gives employees approximately five minutes to relocate outside of the CO2-enriched atmosphere before their 15-minute TWA exceeds the exposure limit. The alarms continue to sound, and the fans continue to run at maximum airflow, until the CO2 concentration is verified to be below the 15,000 ppm STEL for a period of ten minutes, after which the system automatically resets, serving as an all-clear signal.
STEP 5: SUMMARIZE THE ANALYSIS FOR EFFECTIVE STAKEHOLDER COMMUNICATION
The EHS manager for the brewery prepared a summary report describing how the selected instrument was determined to be fit for purpose, what the RTDS was intended to accomplish, and how the alarm setpoints and specific defined responses from the system were established. This report was used to assist with insurability review; it also helps to ensure the system is properly maintained and provides transparency for workers seeking information about how they are protected from hazardous exposures. The summary was also used, in part, during a regulatory inspection and became part of a workers’ compensation claim proceeding. In each instance, the report is valued for providing important institutional memory. Finally, the report is used to determine what upgrades might be needed when a change to the facility is planned.
Ultimately, the decision to archive the data log on a cloud database platform allowed for creation of a substantial training dataset for machine learning evaluation. Following training closely supervised by a qualified data analyst, this dataset is used to help identify gas detection trends that suggest an upcoming period of peak exposure. When the system alerts employees to this potential, controls are enacted and discretionary excursions into the areas of potential concern are rescheduled. This insight also allows for fine-tuning of system gas controls during a system expansion.