DEPARTMENTS
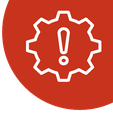
INSTRUMENTATION
SPENCER PIZZANI, CIH, is the occupational health manager for PepsiCo Global EHS.
ROBERT HENDERSON is past chair of the AIHA Real-Time Detection Systems Committee and president of GfG Instrumentation Inc.
STEVEN JAHN, CIH, MBA, FAIHA, is a technical advisor for Savannah River Mission Completion LLC and president of Jahn Industrial Hygiene LLC.
Read the first installment in this series in the June/July 2023 issue.
Send feedback to The Synergist.
Practical Examples of Alarm-Setting Guidance, Part 2
BY SPENCER PIZZANI, ROBERT HENDERSON, AND STEVEN JAHN
Setting alarms for direct-reading instruments (DRI) can be the most complex part of implementing a real-time detection system (RTDS). New guidance available from AIHA (PDF) provides practical guidance for setting alarms, including five steps to determine the appropriate settings based on a systematic analysis of signaling context (see Table 1). The AIHA RTDS Committee is pleased to partner with The Synergist to provide examples of this guidance in action to reinforce practical application. The following scenario concerns the use of four-gas personnel monitors in surface mine blasting.
STEP 1: ESTABLISH STATEMENT OF PURPOSE
A surface mining operation uses a slurry mixed at the blasting hole and injected into a sleeve. During backfilling operations around the sleeve, slurry vapor emissions consisting of nitrogen dioxide (NO2), carbon monoxide, and hydrogen sulfide are purged out of the blasting hole directly into the working zone of the employee placing the charge, who is known in the industry as a “tender.” The mine operates continuously, with blast crews working 12 hours a day and four days in a row. Approximately 150 holes are drilled, and slurry is placed in all prepared holes. The backfilling step lasts for two to three minutes at each hole.
The purpose of establishing gas-specific alarm criteria is to warn the worker to move away from the hole and avoid excessive exposure to slurry vapor emissions. Datalogging functions also allow trending of the occurrence of such alarm conditions to determine supplemental controls such as work behavior modifications or supplemental engineering considerations. The company retained an occupational hygienist to perform this analysis and ensure that the recommended alarm set-points were “fit for purpose” given the selected instrumentation.
STEP 2: SELECT OBJECTIVES
The primary exposure concern is with NO2 due to a very low occupational exposure limit utilized by the company. According to the AIHA alarm-setting guidance, NO2 is a Designation B hazard, which indicates that it has the potential for adverse health effects. The objective of monitoring is to warn the tender to move away when NO2 exposures are detected.
STEP 3: SELECTION OF DEVICES AND DETERMINATION OF FITNESS FOR PURPOSE
The context of the work environment (that is, the number of holes tended in a shift) and the toxicological basis for the OEL are considered in this step.
Table 1. Proposed Steps for Alarm-Setting Process
Tap or click on the table to view a larger version in your browser.
Instrument Performance
Most instruments include filtering of readings near zero to reduce visible fluctuation. The extent of the filtering band is determined by the instrument manufacturer and is based on the properties of the sensor such as signal strength per ppm and the effects of ambient conditions such as humidity and temperature. Most instruments use unfiltered values to calculate peak or ceiling limits, short-term exposure limits (STELs), and time-weighted averages (TWAs).
NO2 is an oxidizing gas. The electrochemical detection reaction converts NO2 into NO and oxygen. NO accumulates in the sensor electrolyte as nitric acid (HNO3).
The sensor in the deployed instrument is systematically examined in accordance with the RTDS fact sheet “Reporting Specifications for Electronic Real Time Gas and Vapor Detection Equipment” (PDF). Because the instrument had sufficient resolution (0.1 ppm), minimum detection limit (0.3 ppm), and accuracy (plus or minus 15 percent), and due to other practical factors, the instrument is deemed fit for purpose. While readings of less than 0.3 ppm are not visible in the display, at 0.3 ppm and above the instrument is able to resolve step-changes in signal strength equal to 0.1 ppm.
Because the worker exposure profile also contains cross-sensitivities that can affect carbon monoxide and hydrogen sulfide monitoring results, analysis of data logs and event logs is determined to be required for proper interpretation. These known interferences are judged to be acceptable because historically collected data showed that these agents were rarely detected. Finally, the temperature and humidity ranges of the sensor cover the anticipated environmental conditions found at the mine.
Datalogging
The instrument records data in an extractable data log. A separate events log records occasions of alarm. Calculated cumulative averages of the full-shift TWA and STEL are logged. The TWA is calculated based on the duration of the shift. The instrument also records discrete events whenever instantaneous values above the programmed alarm thresholds are exceeded. A review of historical logged data indicates that most exposures were below the 12-hour adjusted TWA and STEL values with only rare instances of exceedance.
Regulatory Basis
The mining industry in this example is uniquely regulated by an authority that uses 1994 ACGIH NO2 values (1 ppm TWA and 3 ppm STEL), while the company seeks to follow the most current ACGIH TLV of 0.2 ppm TWA. ACGIH has not established an NO2 STEL. The TLV basis is reported as “lower respiratory tract irritation.” The current TWA limit value was established to protect asthmatics and their occasion in the workforce.
Toxicological Basis
The toxicological basis of lower respiratory tract irritation was used to inform the appropriateness of adjusting the OEL values according to shift length. Adjusting OELs for extended work shifts ensures that the occupational hygienist considers the peak or cumulative body burden and metabolic clearance rate when determining if extended exposures are allowable. A variety of methods exist for adjusting OELs, ranging from simple and conservative (for example, OSHA adjustments in the lead standards and the Brief and Scala approach referenced in the ACGIH TLV documentation) to complex (such as toxicokinetic models requiring biological half-life data). Deciding which method to use is not as simple as choosing the model with the lowest adjusted limit.
For example, according to the Brief and Scala model, the adjusted exposure limit is calculated by means of the following formula, where h equals the number of hours worked per day:
According to this model, for a 12-hour shift, the adjusted TWA TLV limit for NO2 should be reduced to 0.1 ppm.
A different method proposed by Institut de recherche Robert-Sauvé en santé et en sécurité du travail (IRSST), a Canadian OEHS research organization, adjusts OELs for unusual work schedules according to categories determined by potential health impacts of excessive exposure. IRSST classifies NO2 is a Category IV agent, defined as a “substance that produce[s] effects following a short- or long-term exposure” and requiring adjustment by a factor of 0.67 based on a daily shift length of 12 hours and a 48-hour weekly cycle. According to this model, for a 12-hour shift, the TWA TLV limit for NO2 should be reduced to 0.134 ppm (0.2 ppm x 0.67).
Although this method results in a slightly higher adjusted exposure limit than the Brief and Scala model, the occupational hygienist believes it more accurately reflects the toxicological characteristics of the contaminant. There is no reason to use a more conservative model than the already very conservative IRSST guidance. Also, setting the alarms at an even lower value would be difficult given the capabilities of the client’s existing equipment, probably requiring a major capital investment in new equipment—a step the client is willing to take but only if necessary.
STEP 4: ESTABLISHING ALARM SETPOINT AND RESPONSE PROCESS
After discussion, the company determines that the alarm value policy will be established as 95 percent of the shift-length adjusted OEL as a TWA, and one of two possible values: 95 percent of the published STEL or 95 percent of the ACGIH peak exposures guidance.
The company previously established the policy of enforcing an internal 5 percent “safety margin” to prevent exposures very close to the OEL. Following discussion of the adjustment factors, the company adopts the values shown in Table 2.
The high instantaneous alarm is chosen based on NIOSH guidance identifying a lowest observed adverse effect level (LOAEL) of 30 ppm for respiratory irritation and severe cough in volunteers following a 70-minute exposure.
STEP 5: SUMMARIZING THE ANALYSIS FOR EFFECTIVE STAKEHOLDER COMMUNICATION
The analysis presented in steps 1 through 4 is documented in a summary report that is distributed to company and line management and forms the basis for a briefing with staff, blasting crews, and subcontractors. The transparency of the report is key for communication on worker protection and how to respond to alarms. The company also provides this report to the regulator as evidence of ethical and comprehensive examination of the topic given specific regulatory, technology, and field conditions that set the context.
Table 2. Final Alarm Settings
Tap or click on the table to view a larger version in your browser.
RESOURCES
AIHA: “Adjusting OELs for Extended Work Shifts,” AIHce 2001 (presentation by Doug Wylie, June 2001).
IRSST: “Guide for the Adjustment of Permissible Exposure Values (PEVs) for Unusual Work Schedules” (PDF, March 2015).
Journal of Occupational and Environmental Hygiene: “Occupational Exposure Limits: An Approach and Calculation Aid for Extended Work Schedule Adjustments” (December 2005).