Toward a
"
Smart
City"
IH and Security Professionals Collaborate on Early Detection of Bombs
BY SAMANTHA CONNELL
To people working in other fields, industrial hygiene can seem so abstract and confusing that it might be difficult for them to imagine that IH concerns have practical applications in their field. Many top-notch experts work in industrial hygiene, but, as we all know, working on a team can bring fresh ideas to the table, including unrelated ideas that we didn’t see coming. One of the arts of industrial hygiene is making use of new ideas from other fields. Teaming up with other IHs is a great way to develop new ideas, but we can also benefit from working in other fields or merging them with IH.
I was recently able to experience these benefits firsthand while working on a European Union project related to security called Project BONAS: BOmb factory detection by Networks of Advanced Sensors. (Editor's note: This article was written, and this issue of
The Synergist
was in production, prior to the March 22 terrorist attacks in Brussels. For information about the chemical used in the attacks, see the sidebar below.)
PROJECT BONAS
In 2013, an EU regulation on the marketing and use of explosive precursors, EU 98/2013
, limited the concentration of seven substances available to the public (as explained in Annex I of the regulation) and required that eight substances be reported as suspicious transactions when purchased by the public (Annex II). These substances are considered precursors of homemade explosives, or HMEs.
The BONAS project aimed to increase citizen protection and homeland security against terrorist attacks by enabling authorities to thwart bomb factory activity. The goal of Project BONAS was to make possible the early detection of bomb factories by using a network of advanced sensors to detect precursors of HMEs outside of explosives manufacturing sites before
bombs are completed. These sites include any place a bomb is being produced: an apartment, home, warehouse, farm, or other location.
The BONAS project included 13 teams from nine different countries. Our BONAS team was based out of the forensics department of the University of Lausanne (UNIL) in Lausanne, Switzerland, and included three people with starkly different backgrounds: police work, forensics, and analytical chemistry; food chemistry and chemometrics; and industrial hygiene. Throughout the project I worked at a lab belonging to the Institute for Work and Health (IST) in Lausanne—the only IH institute in Switzerland—where I was part of another interdisciplinary group working with partners whose backgrounds included engineering, chemistry, and IH.
The long-term goal of this and similar projects is a “smart city.” Imagine a place with continuous monitoring for hazardous substances (explosive precursors), alerting officials when levels increase from background. This monitoring is accomplished via a network of advanced sensors that are easily camouflaged and deployed in sensitive locations. The sensors are able to identify changes in urban and suburban environments, and can analyze volatile compounds, airborne particle residue, and traces in wastewater. Detecting substances via sensors outside
of manufacturing sites can easily be compared to environmental air sampling.
This is where I enter the picture. My supervisor, Francesco Saverio Romolo, needed someone familiar with air sampling and analysis to complete sampling in parallel with the sensors for validation purposes. He knew that sampling and analytical procedures for precursors of explosives were already developed in industrial hygiene, so he decided to add an IH specialist to his team.
PARALLELS BETWEEN IH AND SECURITY
Many substances of interest to security professionals contribute to common chemical exposures in the workplace. I was familiar with several substances of interest in Project BONAS from previous IH field experience. For example, substances in Annex I of EU 98/2013 include hydrogen peroxide, nitromethane, nitric acid, potassium chlorate, potassium perchlorate, sodium chlorate, and sodium perchlorate. Hexamine, sulfuric acid, acetone, potassium nitrate, sodium nitrate, calcium nitrate, calcium ammonium nitrate, and ammonium nitrate are the substances included in Annex II.
Imagine a place with continuous monitoring for hazardous substances (explosive precursors), alerting officials when levels increase from background.
I referenced several OSHA and NIOSH methods throughout the project, including NIOSH 2549, 1300, and 1501 for volatile organic compounds (VOCs); NIOSH 2527 for nitromethane; and OSHA VI-6 for hydrogen peroxide. In addition, I referenced the German Research Foundation (DFG) and Institute for Occupational Safety and Health of the German Social Accident Insurance (IFA) method 8943 for hydrogen peroxide.
Certain methods proved to be obsolete for our needs due to outdated technology. In some cases, IH methods did not provide a limit of detection low enough to successfully sample in our different project scenarios. For example, OSHA Method ID-126-SG, one of two OSHA methods for hydrogen peroxide, recommends analysis via mercury electrode. This method was not an option for us because mercury electrodes are banned throughout Europe. OSHA Method VI-6 was still available to us, but only partially validated. This left us searching for new methods in the literature.
Some of our project scenarios were more in line with environmental levels, which also have methods available. But the recommended sampling times and flow rates did not agree with our timeline or scenario. For example, the levels of VOCs we were looking for were lower than typical occupational exposure limits, and our desired sampling times were much shorter than environmental sampling times. We needed to adapt these methods to our project. And so, in a quest to find the optimal flow rate for our purposes in one sampling campaign, my colleague Carlotta Ferrari was able to design our experiment using chemometrics. Chemometrics uses mathematical and statistical methods to extract information by analyzing chemical data and optimize procedures or experimental designs. It can also be used to merge data from many different sources. This approach provided the most favorable number of trials needed in which configurations to get the most reliable results in our study.
Development and improvement of sampling and analytical methods is a common goal for both the IH and security fields. In addition to referencing many IH methods, we worked to improve and optimize methods for our specific interests and for the IH field in general. The BONAS and IST groups worked together to adapt and develop two new analytical techniques for identifying hydrogen peroxide during the bomb-making process and, consequently, in the workplace. We used these methods for sampling and analysis during the project. Although the exposure limit for hydrogen peroxide is not extremely low, enhancing the method now allows for short-term sampling. This proved beneficial: new literature suggests a short-term exposure limit (STEL) for this substance, which would not be possible to determine using the current recommended method. In short, both IH and security will benefit from improving the method, even though the side project stemmed from the separate needs of each field.
THE SENSING PERSPECTIVE
Another area where IH and security overlap is sensor technology. Many sensors are developed to identify a substance in a known amount or controlled environment, and difficulties arise when considering how to capture a sample and identify it in a real-world environment. For example, some substances can only be measured as a liquid or solid due to limitations of the technology, or the sampling unit within a sensor isn’t optimized for what the researcher wants to collect. One researcher on the BONAS project was surprised to find that his sampling unit contained media that is very common in the IH field. The IH on the IST team and I were able to clear up some of his questions about the optimal use of the media and other specifications, given our background and expertise in sampling.
Understanding and identifying peak exposures is imperative for preventing both the formation of explosives and adverse health effects to workers. Several activities can occur during the bomb-making process that provide optimal opportunities for authorities to discover the threat. Bomb making involves measuring, pouring, and mixing many different substances of interest. The sensors developed during the BONAS project are intended to alert authorities to heightened levels of these substances. Similarly, we are concerned about activities in the workplace where peak exposures can occur to a worker. However, it is often difficult to capture the profile of a peak exposure when utilizing typical air sampling and analytical methods. For this reason, adapting equipment from other fields could certainly be of interest.
The ability to identify peak exposures individually or via continuous monitoring already exists for multiple chemicals of interest. But new techniques developed by the BONAS and IST groups allow us to conduct reliable short-term sampling and continuous monitoring in the work environment, relaying real-time results. These new methods are the first available for short-term sampling or continuous monitoring for hydrogen peroxide. One new technique uses a method of analysis referred to as “FOX.” It uses a portable photonic detection instrument developed to continuously measure ferrous oxidation in the presence of xylenol orange. This in turns gives us the potential for oxidative stress present in the environment. The other technique developed is a chemiluminescent technique, capable of analyzing directly on-site as well. The outcome of our work shows us that opportunities exist for continuous on-site monitoring via a portable FOX set-up, or simple on-site analysis using a portable luminometer.One researcher on the BONAS project was surprised to find that his sampling unit contained media that is very common in the IH field.
A DUAL OPPORTUNITY
Sensing technology developed for security purposes could potentially be applied in the workplace. Perhaps several of the sensors we used could be implemented for occupational or environmental use. The sensors developed and enhanced throughout the project include technologies such as light detection and ranging (LIDAR)/differential absorption LIDAR detection system (DIAL) sensor; quartz-enhanced photo-acoustic spectroscopy sensor (QEPAS); surface-enhanced Raman spectroscopy sensor (SERS); immunosensors; and electrochemical sensors. A basic illustration of how these sensors can be deployed to identify bomb-making activities is available on the Project BONAS home page
.
Another opportunity is the further development of optimal exposure assessment strategies, potentially bridging the gap between pharmacokinetic modeling, biomarkers, and actual adverse health effects to the worker.
Merging IH with security or other fields could result in many beneficial opportunities. Take our project, for example: our team developed and completed work on improved and novel methods for hygienists in less than a year. Imagine the numerous possibilities that would come with collaboration over time.
FINDING NEW IDEAS
Collaborating with physicists, chemists, and professionals in other fields is important to advancing industrial hygiene. A Google search or literature review can unearth the “latest” technology, but on many occasions, the technology is already developed for another purpose by the time of publication or isn’t very novel anymore.
Industrial hygienists who work on interdisciplinary teams can influence the purpose and use of technology for our specific field early on. I often collaborated with researchers on my team throughout our project to explain what IHs look for in an occupational setting, or to discuss the concerns and realities of certain equipment. In turn, they shared their current capabilities and ideas so that we could decide together on developments that would suit each of our needs. Here’s one example of something we discussed as a team: if analysis can take place on site but requires the use of a liquid media, we may want to adapt the method so that it’s not necessary to require the worker to wear an impinger while still allowing analysis to be completed on site. Researchers may not have experienced impingers in the field on a working body and may not find this to be problematic. But as IHs, we know that this is not the optimal experience for us, the worker, or the equipment.
INTERDISCIPLINARY POTENTIAL
Industrial hygienists are well aware of the benefits of ideas that emerge from working on interdisciplinary teams. This teamwork is vital for the growth and evolution of the IH field, as well as other professions.
Though many companies are dedicated to advancing IH equipment, and technological progress is made all the time, we should continue to work toward building equipment and developing methods to fit our needs rather than adapting existing tools for our purposes. Interdisciplinary teamwork in particular provides a way for people from different fields to conquer their overlapping needs and contribute to their professions—together.
SAMANTHA CONNELL, MSPH,
is an industrial hygienist for Syngenta in Monthey, Switzerland. She can be reached at slconnell89@gmail.com
.Project BONAS is a collaborative project funded under the 7th Framework Programme of the European Commission. For more information, visit the European Commission
website
or the BONAS project home page
.TATP Used in Brussels Attacks
Editor's note: Professor Francesco Saverio Romolo of the Sapienza University of Rome contributed to this report and provided the list of resources below.On March 22, terrorists detonated explosives at the Maalbeek metro station in Brussels, Belgium, and at the Brussels Airport in Zaventem. The attacks killed 32 people and injured more than 300.
As reported in
C&EN
, the explosive used in the attacks was triacetone triperoxide (TATP), a chemical also used in the 2005 bombings in London. According to C&EN
, X-ray computed tomography (CT) and ion mobility spectrometry (IMS) are able to detect TATP, but those methods are useful only when the material is subjected to screening. Portable sensors that can detect TATP in unsecured areas are under development.
The C&EN
article quotes David Atkinson, the head of research in explosives and biological threat detection at Pacific Northwest National Laboratory, as advocating for a smart-city approach to public safety that would attempt to detect “bomb factories” before an attack is underway. Read the C&EN
report online
.
According to the Encyclopedia of Explosives and Related Items
, TATP has never found practical application as a commercial or military explosive because it is too dangerous, due to its tendency to sublimation and its sensitivity to impact, friction, and heating, especially if stored as a dry product. The major threat to security today is that the instructions to synthesize it are easily available.
The rapid detection of uninitiated (pre-blast) TATP can be carried out using tests based on anilinhydrochlorid, N,N-diethyl-p-phenylendiaminesulphate, diphenylamine or thymol, or on the oxidation capability of TATP degradation products, in presence of a peroxidase enzyme, producing a pronounced change of a substrate colour or a luminescence.
Trace recovery, detection and identification of TATP play a critical role in security and criminal investigations. The sampling from smooth surfaces such as luggage in an airport or of items found on the scene of a bombing is well known to industrial hygienists: swabbing. The industrial hygiene community can therefore support the analytical development to find TATP traces, while the security and forensic approaches can be useful for IH applications.
Traces of TATP sampled by swabbing can be analysed by different methods, including gas chromatography/mass spectrometry (GC/MS) and high performance liquid chromatography/mass spectrometry (HPLC/MS).
Resources on TATP
Advances in Analysis and Detection of Explosives, Proceedings of the 4th International Symposium on the Analysis and Detection of Explosives
: “The Identification of Organic Peroxides” (1992).
Analyst
: “Development of an LC/MS Method for the Trace Analysis of Triacetone Triperoxide” (2002).
Analytical and Bioanalytical Chemistry
: “A Quantitative Chemiluminescent Assay for Analysis of Peroxide-based Explosives” (April 2011).
Analytical and Bioanalytical Chemistry
: “Recent Methods for the Determination of Peroxide-based Explosives” (October 2006).
Analytical Chemistry
: “In Situ Trace Detection of Peroxide Explosives by Desorption Electrospray Ionization and Desorption Atmospheric Pressure Chemical Ionization” (March 2008).
Chemische Berichte
: “Über die Einwirkung von Wasserstoff Superoxyd auf Aceton und Mesityloxyd R. 28” (1895).
Encyclopedia of Explosives and Related Items,
Vol. 1 (1960).
Explosives
, 5th ed. (Wiley-VCH, 2002).
Forensic Science International
: “Establishing a Universal Swabbing and Clean-up Protocol for the Combined Recovery of Organic and Inorganic Explosive Residues” (November 2012).
Forensic Science International
: “Headspace GC/MS Detection of TATP Traces in Post-explosion Debris” (December 2004).
Forensic Science International
: “Surface-sampling and Analysis of TATP by Gas Chromatography/Mass Spectrometry” (January 2013).
Journal of Forensic Sciences
: “An Explosive Drug Case” (March 1992).
Journal of Forensic Sciences
: “Improved Method for the Detection of TATP after Explosion” (September 2004).
Journal of Forensic Sciences
: “An Unusual Explosive, Triacetone Triperoxide (TATP)” (July 1986).
Journal of Forensic Sciences
: “Trace Analysis of Peroxide Explosives by High Performance Liquid-Chromatography-Atmospheric Pressure Chemical Ionization-Mass Spectrometry (Hplc-Apci-Ms) for Forensic Applications” (November 2004).
Method and kit for peroxidase detection of peroxide-type concealed explosives, Patent WO 9943846 (1999).
Proceedings of the 1st International Symposium on the Analysis and Detection of Explosives
: “Identification of Two Rare Explosives” (1983).
Propellants, Explosives, Pyrotechnics
: “Determination of the Vapor Density of Triacetone Triperoxide (TATP) Using a Gas Chromatography Headspace Technique” (April 2005).
Rapid Communications in Mass Spectrometry
: “Analysis of Oligomeric Peroxides in Synthetic Triacetone Triperoxide Samples by Tandem Mass Spectrometry” (February 2009).
Rapid Communications in Mass Spectrometry
: “Analysis of Triacetone Triperoxide (TATP) and TATP Synthetic Intermediates by Electrospray Ionization Mass Spectrometry” (January 2008).
Rapid Communications in Mass Spectrometry
: “Analysis of Triacetone Triperoxide by Gas Chromatography/Mass Spectrometry and Gas Chromatography/Tandem Mass Spectrometry by Electron and Chemical Ionization” (October 2006).
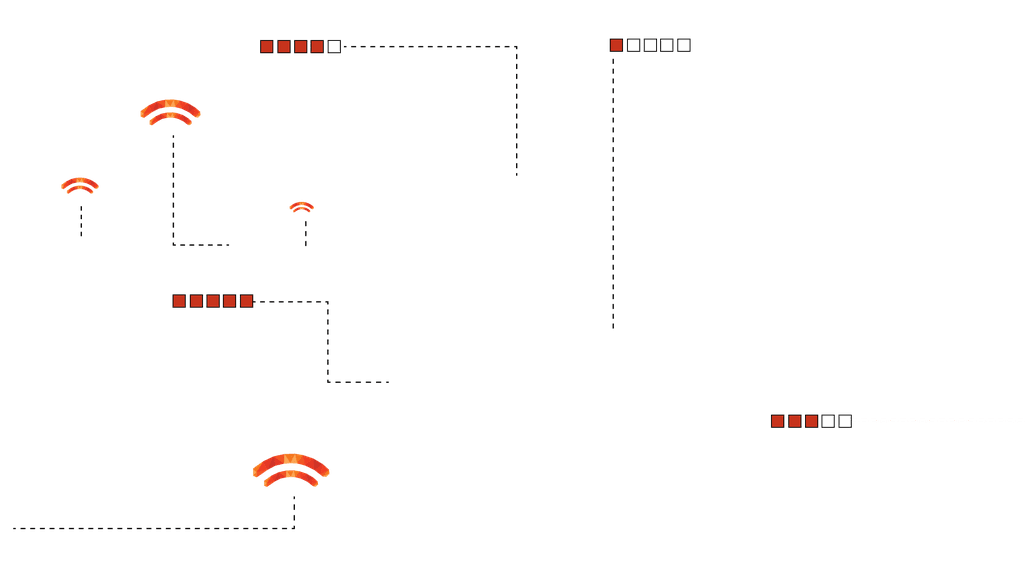
thesynergist | TOC | NEWSWATCH | DEPARTMENTS | COMMUNITY